New
understanding of ocean turbulence could improve climate models
Kevin Stacey, Brown University
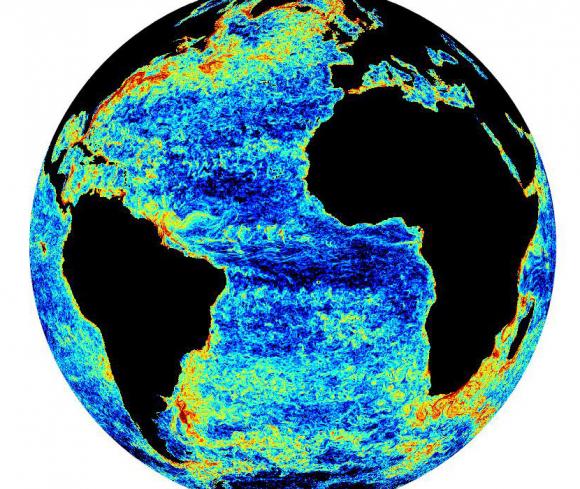
The
study was focused on a form of turbulence known as mesoscale eddies, ocean
swirls on the scale of tens to hundreds of kilometers across that last anywhere
from a month to a year. These kinds of eddies can pinch off from strong
boundary currents like the Gulf Stream, or form where water flows of different
temperatures and densities come into contact.
“You
can think of these as the weather of the ocean,” said Baylor Fox-Kemper,
co-author of the study and an associate professor in Brown’s Department of
Earth, Environmental and Planetary Sciences.
“Like storms in the atmosphere, these eddies help to distribute energy, warmth, salinity and other things around the ocean. So understanding how they dissipate their energy gives us a more accurate picture of ocean circulation.”
“Like storms in the atmosphere, these eddies help to distribute energy, warmth, salinity and other things around the ocean. So understanding how they dissipate their energy gives us a more accurate picture of ocean circulation.”
The
traditional theory for how small-scale turbulence dissipates energy states that
as an eddy dies out, it transmits its energy to smaller and smaller scales. In
other words, large eddies decay into smaller and smaller eddies until all the
energy is dissipated. It’s a well-established theory that makes useful
predictions that are widely used in fluid dynamics. The problem is that it
doesn’t apply to mesoscale eddies.
“That
theory only applies to eddies in three-dimensional systems,” Fox-Kemper said.
“Mesoscale eddies are on the scale of hundreds of kilometers across, yet the
ocean is only four kilometers deep, which makes them essentially
two-dimensional. And we know that dissipation works differently in two
dimensions than it does in three.”
Rather
than breaking up into smaller and smaller eddies, Fox-Kemper says,
two-dimensional eddies tend to merge into larger and larger ones.
“You
can see it if you drag your finger very gently across a soap bubble,” he said.
“You leave behind this swirly streak that gets bigger and bigger over time.
Mesoscale eddies in the global ocean work the same way.”
This
upscale energy transfer is not as well understood mathematically as the
downscale dissipation. That’s what Fox-Kemper and Brodie Pearson, a research
scientist at Brown, wanted to look at with this study.
They
used a high-resolution ocean model that has been shown to do a good job of
matching direct satellite observations of the global ocean system. The model’s
high resolution means it’s able to simulate eddies on the order of 100 kilometers
across. Pearson and Fox-Kemper wanted to look in detail at how the model dealt
with eddy dissipation in statistical terms.
“We
ran five years of ocean circulation in the model, and we measured the damping
of energy at every grid point to see what the statistics are,” Fox-Kemper said.
They found that dissipation followed what’s known as a lognormal distribution —
one in which one tail of the distribution dominates the average.
“There’s
the old joke that if you have 10 regular people in a room and Bill Gates walks
in, everybody gets a billion dollars richer on average — that’s a lognormal
distribution,” Fox-Kemper said. “What it tells us in terms of turbulence is
that 90 percent of the dissipation takes place in 10 percent of the ocean.”
Fox-Kemper
noted that the downscale dissipation of 3-D eddies follows a lognormal
distribution as well. So despite the inverse dynamics, “there’s an equivalent
transformation that lets you predict lognormality in both 2-D and 3-D systems.”
The
researchers say this new statistical insight will be helpful in developing
coarser-grained ocean simulations that aren’t as computationally expensive as
the one used in this study. Using this model, it took the researchers two
months using 1,000 processors to simulate just five years of ocean circulation.
“If
you want to simulate hundreds or thousands or years, or if you want something
you can incorporate within a climate model that combines ocean and atmospheric
dynamics, you need a coarser-grained model or it’s just computationally
intractable,” Fox-Kemper said.
“If we understand the statistics of how mesoscale eddies dissipate, we might be able to bake those into our coarser-grained models. In other words, we can capture the effects of mesoscale eddies without actually simulating them directly.”
“If we understand the statistics of how mesoscale eddies dissipate, we might be able to bake those into our coarser-grained models. In other words, we can capture the effects of mesoscale eddies without actually simulating them directly.”
The
results could also provide a check on future high-resolution models.
“Knowing
this makes us much more capable of figuring out if our models are doing the
right thing and how to make them better,” Fox-Kemper said. “If a model isn’t
producing this lognormality, then it’s probably doing something wrong.”
The
research was supported by the National Science Foundation (OCE-1350795), Office
of Naval Research (N00014-17-1-2963) and the National Key Research Program of
China (2017YFA0604100).